What is Sleep ?
I think all the readers would sleep. Then can you explain what is sleep ? Oh..O. Not easy !! There may not be a single / clear cut definition of sleep even when you ask experts. In this kind of situation, a typical approach is to try to figure out what exactly happens when we sleep. This also may not be easy but everybody would be able to come up with at least a
few observations / experiences.. something like below. Just try to speak out whatever comes up in your mind regardless of whether it is right or wrong
- Close eyes. Really, does everybody closes eyes whenever they sleep ?
- Voluntry Muscle activity reduced. Voluntary muscle activity would not completely shut down, but we may agree that the muscle activity would not be as high as we are awake. Note that the word 'valuntary' is important. That is, the muscle acticity reduction happens to voluntary mustle (like muscles in arms, legs etc) and would not be obvious about non-voluntary muscles like muscles
of heart.
- Go through a specific stage during which eye movement gets very rapid. This is called REM(Rapid Eye Movement) stage, but I don't think you feel / observe this on your own. Does every sleeping animal through this REM stages ?
- Shows a specific brainwave patterns
In this note, I will talk about various aspect of the activities during sleep and followings are the list of topics to be covered.
Does every animal sleep ? Scientists believe all animal sleep. but the duration and patterns of sleep can vary greatly among different species. Some animals, such as dolphins and certain birds, are able to enter a state of rest called unihemispheric slow-wave sleep, in which only one half of their brain sleeps at a time, allowing them to continue to swim or fly and remain alert for potential predators. Other animals, such as bats, can enter into a state of torpor, which is a form of hibernation-like
sleep that allows them to conserve energy during periods of food scarcity.
We would know that higher animals like mammals would sleep just by direct observation. But there can be various cases where it is hard to know whether a specific animals sleep or not. However it is known by scientists that even very simple animal like Hydra sleeps.
Sleep is so essential that some animals sleeps only in a part of the brain when they cannot sleep with both brains. For example, a seal while floating on the sea it cannot go to sleep with the whole brain since it will drown to death if they sleep with the whole brain. In this csae, they sleep in only half of the brain
How do you know if an animal sleep ? If the animal is a human, it is relatively easy to figure out whether they are sleep or not. You would know how to know for human without any further explanation excep for some rare case for those who sleep without closing eyes or those sleeping sitting in chair without closing eyes etc :).
Followings are some of the most common indicator to checkif an animal is in sleep or not.
- Neural changes like Brain wave changes
- Physiological changes like changes in a certain hormones
- Checking the response to stimulus
- Observing the activity
If you are a researcher in this area, the best way would to be use various measurement equipment like EEG(ElectroEncelphalGram), EMG (ElectroMyoGram), EOG(Electro-oculography machine) etc. You may use these method for most of higher animals like mammal, birds and even for some reptiles (like crocodiles).
How about for lower animals like Fish, Hydra etc ? In those case, researchers mostly use the activity (e.g staying still without moving) or responses to stimulus as indicators for sleep.
Researchers have observed that even single-celled organisms, such as some species of protozoa, display patterns of activity and inactivity that are consistent with a sleep-like state. In more complex animals, scientists have used a variety of methods to study the neural and physiological changes that occur during sleep, such as monitoring brain activity, measuring changes in hormone levels, and observing changes in muscle tone and movements. Additionally, studies have also been conducted to
see the consequences of deprivation of sleep, which have shown that sleep deprivation can have negative effects on the behavior, cognitive abilities, and overall health of animals.
Why all animal sleep ? (at least, why all animal seems to sleep ?). In short, we don't have any clear answer that fit for all animals. We are just making guesses from various researches and making a list of possible reasons for sleep. Some of the most commonly mentioned reason for sleep are as follows.
- Memory Consolidation
- Reducing Energy Consumption and reserving energies for other vital functionalities
- Cleaning up the metabolic waste (e.g, Cleaning up Glymphatic System) - Why Sleep is Important
Following is an example on how sleep impact on Memory Process. As shown in [a], you would notice :
- You would have higher recognition performance as you have longer slow-wave-sleep (NOTE: slow-wave-sleep implies deeper sleep. Refer to the note on sleep cycle to get further details on sleep cycle).
- Memory process improvement with sleep is observed more drastically for remote memory (NOTE : recent memory refer to the memory formed recently and remote memory refer tos the memory formed relatively long time ago).
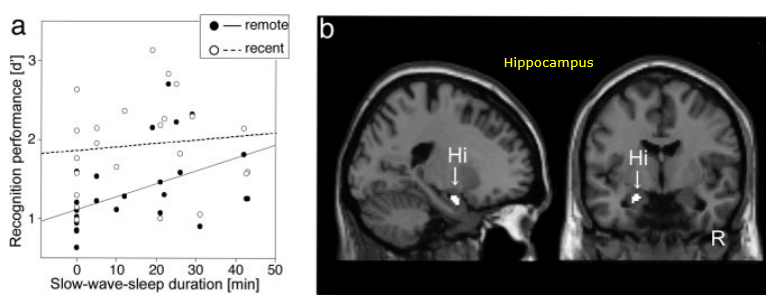
Image Source : Declarative memory consolidation in humans: A prospective functional magnetic resonance imaging study
In following research, radio labelled chemicals (Amyloid Beta, Inuline) are injected in prefrontal cortex of mice in awake, sleep and anesthetized state and measured how fast those chemicals cleared out of the brain. Brains were harvested 10 to 240 min later for analysis.
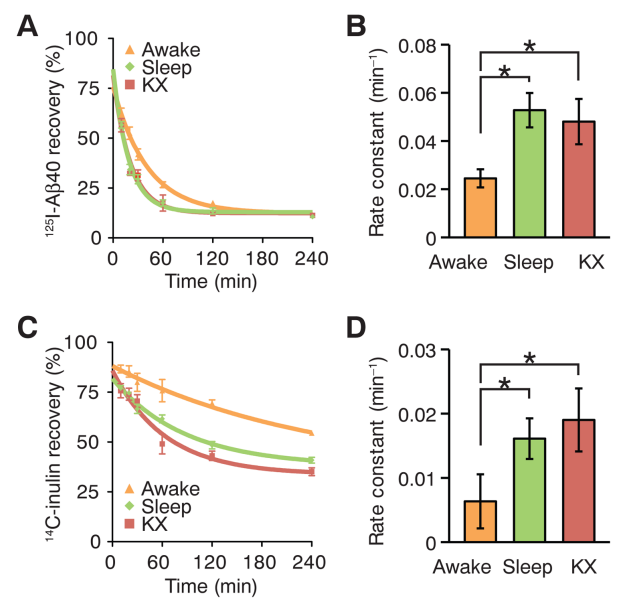
KX : anesthetized with ketamine/xylazine
Image Source : Sleep Drives Metabolite Clearance from the Adult Brain
[A],[C] shows the recovery late over time. Lower value indicates that more of the materials has been washed out (cleared)
[B],[D] shows the rate of the clearance. Higher value indicates that the materila is washed our more quickly.
The result shows that both Amyloid Beta and Inuline gets washed out (cleared) more rapidly during sleep or anesthetized than awake.
NOTE : Amyloid beta
Amyloid beta is a peptide that is involved in the development of Alzheimer's disease, a neurodegenerative disorder characterized by memory loss and cognitive decline. Amyloid beta is a protein fragment that is produced by the abnormal cleavage of a larger protein called amyloid precursor protein (APP). In Alzheimer's disease, amyloid beta peptides tend to aggregate and form plaques in the brain, which are thought to contribute to the death of nerve cells and the development of the disease.
Research has shown that brain energy consumption during sleep is generally lower than during wakefulness.
One study using positron emission tomography (PET) imaging found that during NREM sleep, brain glucose metabolism (a measure of brain energy consumption) is about 6-8% lower than during wakefulness. During REM sleep, brain glucose metabolism is about 10-20% lower than during wakefulness. Additionally, a study using functional magnetic resonance imaging (fMRI) found that during NREM sleep, brain activity in the prefrontal cortex, which is involved in higher cognitive functions such as attention
and decision making, is significantly reduced compared to wakefulness.
Following figure shows energy consumption changes in brain during wake and sleep stage
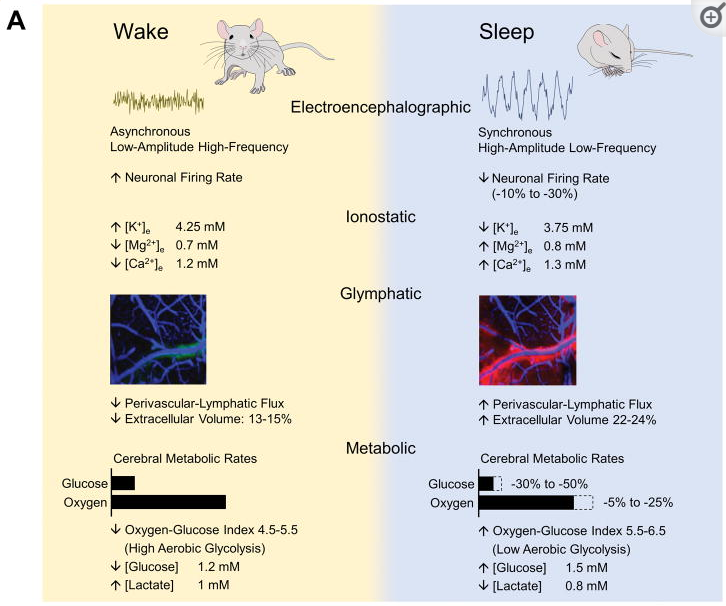
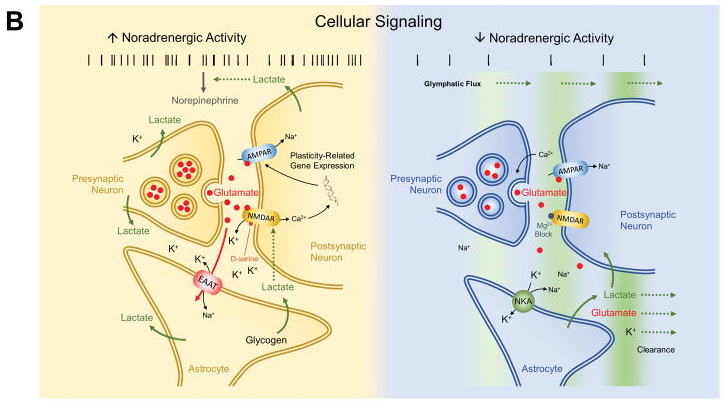
Image Source : Brain Energetics During the Sleep-Wake Cycle
Following figure shows energy consumption changes and a few others in overall body part during wake and sleep stage
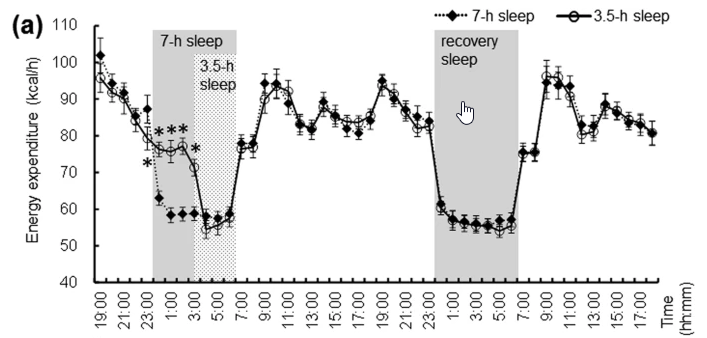
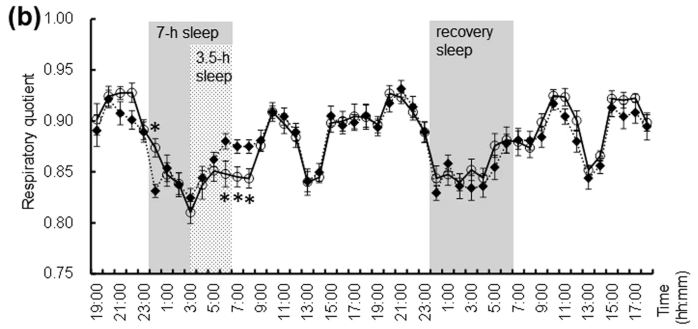
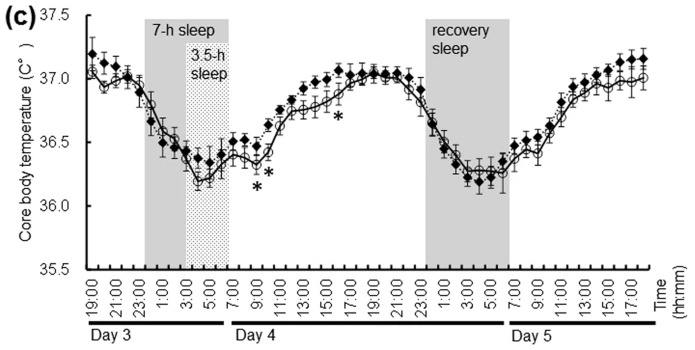
Image Source : Effect of shortened sleep on energy expenditure, core body temperature, and appetite: a human randomised crossover trial
Not all animals sleep for the same amount of time. Some animals like elephants barely sleep, while others like bats can snooze for almost the whole day. It can also depend on the animal, like horses sleep standing up and dolphins sleep for short intervals alternating one side of their brain and the other side. Even within the same species, it can vary depending on the age or health of the animal. Like baby animals tend to sleep more than grown-ups and sick animals may sleep more than healthy
ones.
Table Source : How Much Do Animals Sleep?
Species |
Average Total Sleep Time (% of 24 hr) |
Average Total Sleep Time (Hours/day) |
Brown Bat |
82.9% |
19.9 hr |
Giant Armadillo |
75.4% |
18.1 hr |
North American Opossum |
75% |
18 hr |
Python |
75% |
18 hr |
Owl Monkey |
70.8% |
17.0 hr |
Human (infant) |
66.7% |
16 hr |
Tiger |
65.8% |
15.8 hr |
Tree shrew |
65.8% |
15.8 hr |
Squirrel |
62% |
14.9 hr |
Western Toad |
60.8% |
14.6 hr |
Ferret |
60.4% |
14.5 hr |
Three-toed Sloth |
60% |
14.4 hr |
Golden Hamster |
59.6% |
14.3 hr |
Platypus |
58.3% |
14.0 hr |
Lion |
56.3% |
13.5 hr |
Gerbil |
54.4% |
13.1 hr |
Rat |
52.4% |
12.6 hr |
Cat |
50.6% |
12.1 hr |
Cheetah |
50.6% |
12.1 hr |
Mouse |
50.3% |
12.1 hr |
Rhesus Monkey |
49.2% |
11.8 hr |
Rabbit |
47.5% |
11.4 hr |
Jaguar |
45% |
10.8 hr |
Duck |
45% |
10.8 hr |
Dog |
44.3% |
10.6 hr |
Bottle-nosed dolphin |
43.3% |
10.4 hr |
Star-nosed Mole |
42.9% |
10.3 hr |
Baboon |
42.9% |
10.3 hr |
European Hedgehog |
42.2% |
10.1 hr |
Squirrel Monkey |
41.3% |
9.9 hr |
Chimpanzee |
40.4% |
9.7 hr |
Guinea Pig |
39.2% |
9.4 hr |
Human (adult) |
33.3% |
8 hr |
Pig |
32.6% |
7.8 hr |
Guppy (fish) |
29.1% |
7 hr |
Gray Seal |
25.8% |
6.2 hr |
Human (elderly) |
22.9% |
5.5 hr |
Goat |
22.1% |
5.3 hr |
Cow |
16.4% |
3.9 hr |
Asiatic Elephant |
16.4% |
3.9 hr |
Sheep |
16% |
3.8 hr |
African Elephant |
8.3% |
2.0 hr |
Donkey |
13.0% |
3.1 hr |
Horse |
12.0% |
2.9 hr |
Giraffe |
7.9% |
1.9 hr |
A sleep cycle is a recurring period of sleep during the night. The typical sleep cycle lasts around 90 minutes and includes several distinct stages of sleep, including light sleep, deep sleep, and rapid eye movement (REM) sleep. During the cycle, the brain and body progress through these stages in a predictable pattern, with the amount of time spent in each stage varying depending on the stage of the cycle. The first sleep cycles of the night typically have longer periods of deep sleep, while
later cycles have more REM sleep. The overall length of the sleep cycle can vary depending on the individual and their sleep needs
Following diagram shows the overall sleep cycle we go through (assuming that we have several hours of good sleep :). Giving you just a big picture of this diagram :
- Each of the cycles spans around 60 to 90 mins
- The first two cycles goes through the deepest cycle (i.e, up to Stage 4).
- At some point of most cycles, we go through REM(Rapid Eye Movement) sleep
- (In general) As we go through deeper stages of sleep, the frequency of the brain tend to get lower (i.e, slower brain wave)
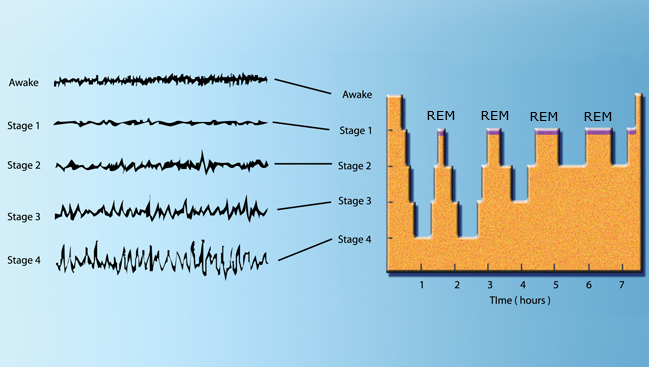
Image Source : Brain Activity During Sleep
Following shows the changes of EEG(brain wave), EMG(Muscle Activity), EOG(Eye Movement) during the sleep cycle.
- EEG Changes in Sleep Stages
- During NREM sleep(Stage 1,2,3,4), EEG readings show slower, higher amplitude brain waves, while during REM sleep, EEG readings show faster, lower amplitude brain waves
- Among the stages during NREM sleep, EEG reading shows slower and higher emplitude as the stage goes deeper.
- EMG Changes in Sleep Stages
- During NREM sleep, muscle activity continues to decrease as the person progresses through the stages of N2 and N3. In N2, muscle activity is more synchronized, while in N3, muscle activity is at its lowest level.
- During REM sleep, EMG readings show low levels of muscle activity, which is known as atonia. This state of muscle relaxation is thought to prevent the person from acting out their dreams, which can be vivid and sometimes disturbing.
- EOG Changes in Sleep Stages
- During NREM sleep, eye movement continues to decrease as the person progresses through the stages of N2 and N3. In N2, eye movement is less frequent, while in N3, eye movement is almost absent.
- During REM sleep, EOG readings show rapid eye movement (REM) as the eyes rapidly move in different directions. This is a characteristic of the REM stage, where vivid and sometimes disturbing dreams occur.
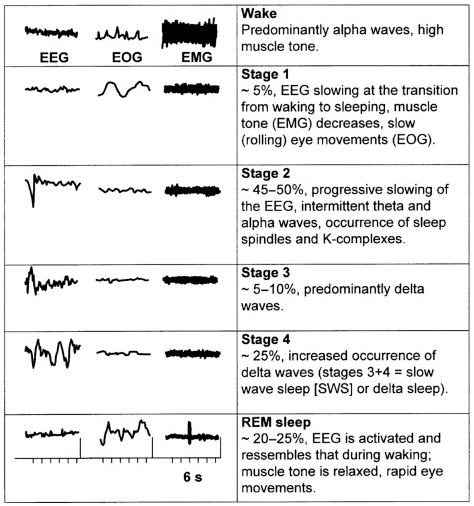
Image Source : Sleep Disorders: Psychiatric Aspects
EEG : ElectroEnchphalogram
EMG : ElectroMyogram
EOG : Electro-oculography
The sleep cycle is often divided into 5 stages as follows. Try to correlate this description with the illustration shown above and then try to memorize illustrations rather than memorize the verbal description if you have more of vision oriented brain like me.
- N1 : Stage 1 (light sleep). Stage N1, also known as drowsy sleep or light sleep, is the stage between being fully awake and falling asleep. During this stage, the brain produces alpha and theta waves, which are slower and of lower amplitude than the beta waves produced during wakefulness. Muscles begin to relax and the person may experience hypnic jerks or sensations of falling. The eyes are closed but the person is easily awakened. This stage usually lasts for 5-10 minutes
- N2 : Stage 2 (light sleep). Stage N2, also known as light sleep, is the first stage of true sleep. The brain produces sleep spindles and K-complexes, which are brief bursts of activity that help to protect the sleeper from external stimuli. The person's heart rate and breathing begin to slow down, and the body temperature drops. This stage usually lasts for around 20-25 minutes and it makes up around 50-55% of an adult's sleep time. It is believed that Sharp Wave and Riiples (SWR) primarily occurs at this stage.
- N3 : Stage 3 (deep sleep). Stage N3 is also known as slow-wave sleep (SWS) because of the slow, large amplitude brain waves that are present during this stage.
- N4 : Stage 4 (deep sleep). N4 is a stage of deep sleep that is also sometimes referred to as delta sleep. It is characterized by even slower brain waves, called delta waves, which can reach as low as 0.5 Hz. This stage of sleep is considered the most restorative and restful stage of sleep, and it is during this stage that the body releases growth hormone and repairs muscles and tissues. During stage N4, it's hard to wake up a person, and if they are woken up, they will
feel
groggy and disoriented.
- REM (Rapid Eye Movement). As the name suggests, REM sleep is characterized by rapid, random movement of the eyes. The detailed activities during REM sleep is described as below.
- Low muscle tone: During REM sleep, muscle tone decreases, leading to a temporary paralysis of the body's skeletal muscles, known as atonia. This is thought to prevent the person from acting out their dreams.
- Increased heart rate and breathing: Heart rate and breathing increase during REM sleep, which may be related to the increased brain activity that occurs during this stage.
- Brain activity: REM sleep is characterized by increased brain activity in the areas of the brain responsible for processing emotions, memories and learning.
- Dreams: REM sleep is associated with vivid and sometimes disturbing dreams
The main difference between N1 and N2 is the level of brain activity and how easily the person can be awakened. The person is in a deeper sleep in N2 than N1 and it's harder to wake them up. The body is also in a more relaxed state during N2 than N1.
Brain activity during NREM sleep, which includes stages N1, N2 and N3, is characterized by slower, synchronized electrical activity, while during REM sleep, the brain's electrical activity is more similar to that seen during wakefulness. During NREM, the brain is less responsive to external stimuli and the body is relatively still, while during REM, the brain is more active, with rapid eye movements, increased heart rate and respiration, and muscle paralysis. The majority of dreaming occurs
during REM sleep, that's why it's also called as "Dream Sleep".
We all have this question, but there is no single clear-cut answers that is agreed by everybody. In this case, the best way would be to brainstorm. Let's review various views on What is dream and why we dream from ancient through modern science. Here goes the list and summary.
Ancient civilizations: In ancient times, dreams were often seen as messages from the gods or as prophecies. In ancient Egypt, dreams were considered divine and were recorded in hieroglyphs. Ancient Greeks, such as Aristotle and Hippocrates, believed that dreams provided insights into an individual's physical and emotional health. The Romans also valued dreams for their potential to provide guidance and foresight.
Medieval period: During the Middle Ages, dreams were often viewed through a religious lens. Christian theologians saw dreams as a form of divine communication or as temptations from the devil. In the Islamic tradition, dreams were considered a source of guidance, with some dreams believed to be direct revelations from God.
Enlightenment and the rise of psychology: With the advent of the Enlightenment and the scientific revolution, dreams began to be studied more systematically. Sigmund Freud's psychoanalytic theory proposed that dreams were a window into the unconscious mind, serving as a way to process unresolved conflicts and repressed desires. Carl Jung, another influential psychologist, believed that dreams could provide access to a collective unconscious, which contained archetypal symbols shared
across human cultures.
Modern neuroscientific view: In modern science, many researchers put forth many different views on this. Some of the modern theory are as follows. To me, every perspective make sense and seems matching to my personal experience and observation on myself.
- Activation-synthesis hypothesis : According to this theory, dreams are a byproduct of random neural activity during the rapid eye movement (REM) stage of sleep, and the brain's higher cognitive centers attempt to make sense of this random activity by synthesizing it into coherent dream narratives.
- Continual-activation theory: According to this theory, dreaming is a result of the brain's need to continually activate and consolidate memories, even during sleep. According to this view, dreams help the brain to integrate new experiences with existing memories, facilitating learning and adaptation.
- Threat simulation theory: According to this theory, dreams serve an evolutionary purpose by simulating threatening situations, allowing individuals to practice and refine their responses to danger. This rehearsal of potential threats could have provided an adaptive advantage for our ancestors, increasing their chances of survival in the face of real-world dangers.
- Information processing and problem-solving theory: According to this theory, dreaming serves to process and organize the vast amount of information encountered during waking hours. Dreams may provide a space for the brain to work through complex issues and find novel solutions to problems, contributing to cognitive flexibility and creative thinking.
- Emotional regulation theory: According to this theory, the role of dreams is processing and regulating emotions. During dreaming, the brain may replay emotionally charged experiences or simulate hypothetical scenarios to help individuals come to terms with their feelings and integrate them into their self-concept.
- Memory consolidation theory: According to this theory, dreaming plays a vital role in consolidating and strengthening memories, particularly those associated with newly acquired skills or emotionally salient experiences. During sleep, the brain selectively rehearses and reorganizes memory traces, which can lead to improved recall and retention.
- Restorative theory: According to this theory, dreaming serves a restorative function, allowing the brain and body to recuperate from the stresses of daily life. Dreaming may help to restore emotional and cognitive balance, promoting overall well-being and mental health.
- Social simulation theory: According to this theory, dreams provide a safe environment for individuals to simulate and rehearse social situations, allowing them to develop and maintain social skills. This perspective emphasizes the importance of social interactions for human well-being and suggests that dreaming may serve an adaptive function in fostering social competence.
- Neurocognitive model of dreaming: According to this theory, dreams are generated by the same neural and cognitive processes responsible for waking thought, with the primary difference being the absence of external sensory input and the inhibition of motor output during sleep.
- Default network activation theory: According to this theory, dreams may be related to the activation of the brain's default mode network (DMN) during sleep. The DMN is a network of interconnected brain regions that become active when an individual is not focused on the external environment or engaged in goal-directed tasks. The activation of the DMN during sleep may contribute to the generation of dream experiences, particularly those involving self-referential and introspective
content.
- Dream-lag effect: This phenomenon suggests that there may be a temporal delay between waking experiences and their incorporation into dreams. The dream-lag effect proposes that events and experiences from waking life may be incorporated into dreams after a delay of several days, potentially reflecting the brain's ongoing process of memory consolidation and integration.
- Expectation-fulfillment theory: According to this theory, dreams allow individuals to fulfill unmet emotional needs or expectations from waking life. According to Griffin, dreaming serves as a kind of emotional "pressure release valve," allowing individuals to resolve emotional tensions and restore psychological balance.
Even though we have gained significant insights into the process, we still don't have a complete understanding of how the brain constructs them. In other words, the exact mechanism of dream construction still not clear. Tt likely involves a combination of neural activity, memory processing, emotional regulation, and cognitive functions
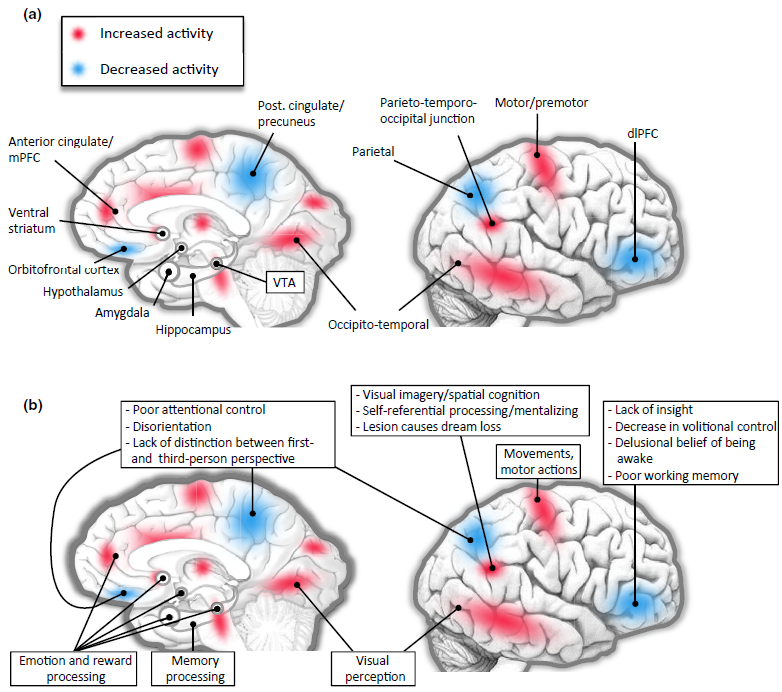
Image Source : Dreaming, Neural Basis of
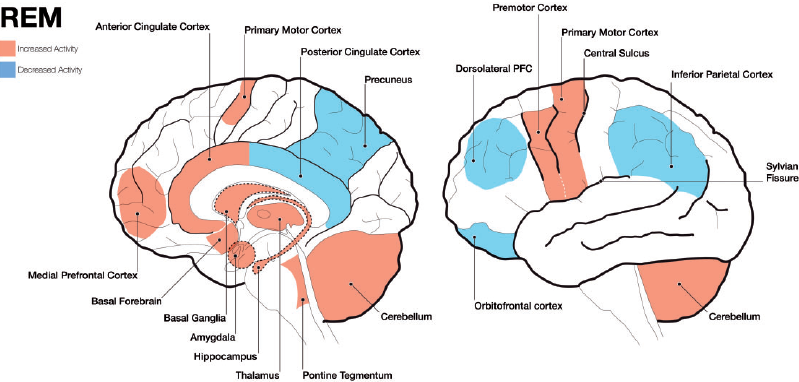
Image Source : Exploring the neural correlates of dream phenomenology and altered states of consciousness during sleep
First, let's take a look at various parts of the brain that is considered to get involved in dreaming (construction of a dream).
- Pons: Located in the brainstem, the pons plays a crucial role in the initiation of REM sleep, when most vivid dreaming occurs. It sends signals to the cerebral cortex, which is responsible for higher cognitive functions such as thought and memory.
- Thalamus: The thalamus acts as a relay station, transmitting sensory and motor signals to the cerebral cortex. During REM sleep, the thalamus becomes more active and may contribute to the vivid sensory experiences in dreams.
- Cerebral cortex: The cerebral cortex is responsible for higher cognitive functions such as thought, memory, and perception. During dreaming, various regions of the cortex are involved in processing and integrating the dream content, including the sensory experiences, emotions, and memories. The temporo-parietal junction, visual association areas, and limbic regions of the cerebral cortex are particularly involved. These areas contribute to the integration of sensory experiences,
emotions, and memories within the dream narrative.
- Amygdala and hippocampus: These structures, part of the limbic system, play key roles in emotional processing and memory consolidation. They are highly active during REM sleep, which may contribute to the emotional content and incorporation of memories in dreams.
- Prefrontal cortex: The prefrontal cortex is associated with executive functions such as decision-making, self-awareness, and social cognition. During REM sleep, activity in some areas of the prefrontal cortex is reduced, which may explain why dreams often lack logic, coherence, and self-awareness.
How these parts are interplaying each other to form a dream ? This mechanism is not clearly established either and would vary depending on different theories/Hypothesis. Followings are some of the example of those theories /Hypothesis :
- Activation-Synthesis Hypothesis: Proposed by J. Allan Hobson and Robert McCarley, this theory suggests that dreaming is the result of the brain's attempt to synthesize and interpret random neural activity during REM sleep. The pons generates random signals, which are relayed to the thalamus and then to the cerebral cortex. The cortex, in turn, tries to create a coherent narrative out of these random signals, integrating memories, emotions, and sensory experiences. The amygdala
and
hippocampus contribute emotional content and memories to the dream narrative.
- Continual-Activation Theory: This theory proposes that dreaming is a byproduct of the brain's continual activation during sleep. According to this view, the brain remains active during sleep to consolidate memories and perform other restorative functions. The interplay among the various brain regions, including the cerebral cortex, thalamus, amygdala, hippocampus, and pons, results in the construction of dreams as a side effect of these ongoing processes.
- Memory Consolidation and Emotional Regulation: Some researchers believe that dreaming is related to memory consolidation and emotional regulation processes that occur during sleep. In this view, the interaction among the hippocampus, amygdala, and various cortical regions facilitates the integration of new information, the processing of emotions, and the strengthening of neural connections. Dreams may emerge as a byproduct of these processes, incorporating memories, emotions,
and
cognitive functions.
Bad dreams or nightmares can result from several factor. Even though occasional bad dreams are normal, frequency and repeating nightmares can be disruptive to sleep quality and may require professional consultation for proper evaluation and treatment.
Followings are some of the factors that is consdered to get involved in bad dream :
- Emotional stress: Negative emotions, such as fear, anxiety, or sadness, can trigger bad dreams, as the brain processes these emotions during sleep.
- Traumatic experiences: Individuals who have experienced trauma may have nightmares as a way for the brain to process and cope with the event.
- Daily experiences: Negative or challenging experiences during the day can be incorporated into dreams and lead to bad dreams.
- Sleep disorders: Conditions like sleep apnea, restless leg syndrome, or insomnia may contribute to the occurrence of nightmares.
- Medications and substances: Some medications or substances, such as alcohol or drugs, can affect brain chemistry and lead to bad dreams.
NOTE : Some examples of medications that might cause bad dream
Certain medications can potentially cause nightmares or vivid dreams as a side effect. Here are some common examples:
- Beta-Blockers: Used to treat high blood pressure and heart conditions, drugs like propranolol can lead to more intense dreams and nightmares.
- Chantix (Varenicline): This medication, used to help people stop smoking, is known for causing vivid, unusual, or disturbing dreams.
- Antidepressants: SSRIs (such as fluoxetine and sertraline) and SNRIs (such as venlafaxine) often affect sleep patterns and can cause vivid dreams or nightmares.
- Sedative-Hypnotics: Medications like zolpidem (Ambien) that are used to treat insomnia can cause unusual dreams and nightmares.
- Antibiotics: Certain antibiotics, such as ciprofloxacin or levofloxacin, have been reported to cause disturbing dreams in some cases.
- Parkinson’s Disease Medications: Drugs used to manage Parkinson’s, including levodopa, can cause vivid dreams and nightmares.
Certain medications can lead to bad dreams or nightmares as a side effect. The exact mechanism by which these medications cause bad dreams can vary depending on the drug and the individual's unique brain chemistry. In general, medications can influence sleep patterns, sleep architecture, and neurotransmitter levels, which can result in vivid, intense, or disturbing dreams
- Antidepressants: Selective serotonin reuptake inhibitors (SSRIs) and tricyclic antidepressants can alter brain chemistry, affecting the balance of neurotransmitters, which may lead to vivid or disturbing dreams.
- Beta-blockers: These medications are used to treat high blood pressure, migraines, and other heart-related conditions. They can affect REM sleep and alter the brain's neurotransmitter levels, potentially causing nightmares.
- Antihistamines: Some antihistamines, especially those with sedating effects, can influence sleep architecture and lead to more intense dreams or nightmares.
- Sleep aids: Some sleep medications, such as benzodiazepines and non-benzodiazepine hypnotics, can alter sleep patterns and increase the likelihood of vivid dreams or nightmares.
- Antipsychotics: These medications can affect the balance of dopamine and other neurotransmitters in the brain, which may lead to vivid or disturbing dreams.
- Cholinesterase inhibitors: Used to treat Alzheimer's disease, these medications can increase acetylcholine levels in the brain and may contribute to vivid dreams or nightmares
there are specific regions in the brain that play a crucial role in controlling the sleep-wake cycle. These brain structures work in conjunction with neurotransmitters to regulate sleep and wakefulness.
Before reading the description, I would suggest you to get familiar with location of each brain parts illustrated below.
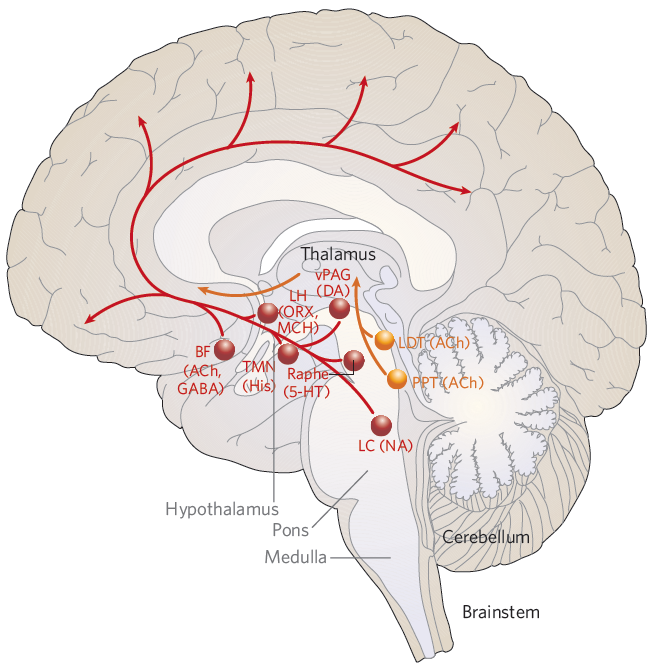
Image Source : Hypotalamic regulation of sleep and circadian rhythms - Research Gate
Some of the key areas include:
Suprachiasmatic nucleus (SCN): Located in the hypothalamus, the SCN serves as the master circadian clock. It receives input from the retina about light levels and adjusts the body's internal clock accordingly. The SCN controls the release of melatonin from the pineal gland and regulates various physiological processes, such as body temperature and hormone release, to align with the sleep-wake cycle. The SCN is responsible for synchronizing various physiological processes with the
24-hour
day-night cycle. It receives light information from the retina and uses this information to regulate melatonin production by the pineal gland, which helps signal the onset of sleep. The SCN also influences other brain regions involved in sleep regulation, such as the ventrolateral preoptic nucleus (VLPO), and coordinates the release of hormones and changes in body temperature to align with the sleep-wake cycle.
Ventrolateral preoptic nucleus (VLPO): Also located in the hypothalamus, the VLPO is responsible for promoting sleep. It contains neurons that release inhibitory neurotransmitters, such as GABA and galanin, which inhibit the activity of wake-promoting regions in the brain.
Locus coeruleus (LC): This area, located in the brainstem, is the primary source of norepinephrine in the brain. The LC is involved in arousal, attention, and vigilance. During sleep, the activity of LC neurons decreases, allowing for the onset and maintenance of sleep.
Tuberomammillary nucleus (TMN): The TMN, located in the hypothalamus, is the primary source of histamine in the brain. Histamine promotes wakefulness, and the activity of TMN neurons is highest during wakefulness and decreases during sleep.
Raphe nuclei: Located in the brainstem, these nuclei are the primary source of serotonin in the brain. Serotonin is involved in regulating mood, appetite, and sleep. Serotonin-producing neurons in the raphe nuclei are less active during REM sleep.
Lateral hypothalamus: This region contains neurons that produce orexin (also known as hypocretin), a neurotransmitter that promotes wakefulness and regulates the sleep-wake cycle. Dysfunction of orexin-producing neurons has been implicated in sleep disorders like narcolepsy.
There are several neurotransmitters that play a role in promoting sleep and inducing sleepiness. Some of the well known neurotransmitter of this kind are as follows. These neurotransmitters and substances work together in a complex interplay to regulate sleep and wakefulness. Their balance and interaction determine the transitions between sleep and wake states.
Gamma-aminobutyric acid (GABA): GABA is the primary inhibitory neurotransmitter in the central nervous system. It helps reduce neuronal excitability and promotes relaxation, which contributes to the onset of sleep. Many sleep-inducing medications, like benzodiazepines and non-benzodiazepine hypnotics, work by enhancing the effects of GABA.
Adenosine: Adenosine is a neuromodulator that accumulates in the brain during wakefulness and promotes sleepiness. As the day progresses, adenosine levels rise, increasing the pressure to sleep. Caffeine and other stimulants counteract sleepiness by blocking adenosine receptors.
Melatonin: Melatonin is a hormone produced by the pineal gland that helps regulate the sleep-wake cycle. Its production is triggered by darkness and inhibited by light. Melatonin levels typically rise in the evening, signaling the body that it is time to sleep, and decrease in the early morning hours.
Prostaglandin D2: This lipid-derived signaling molecule has been shown to promote sleep by acting on certain receptors in the brain. Prostaglandin D2 levels increase during sleep, particularly during non-rapid eye movement (NREM) sleep.
Galanin: Galanin is a neuropeptide that contributes to the regulation of sleep. It is released by neurons in the ventrolateral preoptic nucleus (VLPO), a sleep-promoting region in the brain. Galanin has inhibitory effects and helps suppress the activity of wake-promoting regions.
There are many medications/substances used to promote sleep in people with insomnia or other sleep disturbances. They work by interacting with various neurotransmitter systems in the brain, which regulate the sleep-wake cycle and promote relaxation. Some of the examples are as follows :
Benzodiazepines: These drugs, such as diazepam (Valium) and lorazepam (Ativan), work by enhancing the effects of the inhibitory neurotransmitter gamma-aminobutyric acid (GABA). GABA reduces neuronal excitability, leading to calming and sedative effects that help induce sleep.
Non-benzodiazepine hypnotics: Also known as "Z-drugs," this class includes zolpidem (Ambien), zaleplon (Sonata), and eszopiclone (Lunesta). They also enhance GABA's effects but are more selective in targeting specific GABA receptors, resulting in fewer side effects and a lower risk of dependency compared to benzodiazepines.
Melatonin receptor agonists: Drugs like ramelteon (Rozerem) work by mimicking the effects of melatonin, a hormone produced by the pineal gland that regulates the sleep-wake cycle. This class of medications can help regulate sleep patterns and promote the onset of sleep.
Antidepressants: Some antidepressants, such as trazodone and mirtazapine, have sedative effects and can be prescribed off-label for insomnia. They work by modulating various neurotransmitters, like serotonin and norepinephrine, which can impact sleep.
Antihistamines: Over-the-counter sleep aids often contain antihistamines, such as diphenhydramine (Benadryl) or doxylamine (Unisom). These drugs block the action of histamine, a neurotransmitter involved in regulating wakefulness, resulting in drowsiness.
NOTE : Why I get sleepy when I got medication for cold ? Some medication (Not all), like Night time pill for cold, would make you sleepy. In many cases, this kind of medicine would related to the effect of antihistamine. When you have a cold or flu, your body produces more histamine, which can contribute to symptoms like sneezing, runny nose, and itching. Antihistamines help alleviate these symptoms by blocking histamine's effects. However, since histamine also plays
a role
in regulating wakefulness, blocking its action can lead to drowsiness. Some common antihistamines found in cold and flu medications include diphenhydramine (Benadryl), chlorpheniramine (Chlor-Trimeton), and doxylamine (found in some NyQuil formulations). These antihistamines are known as first-generation antihistamines, which tend to cause more drowsiness than newer, second-generation antihistamines like cetirizine (Zyrtec), fexofenadine (Allegra), and loratadine (Claritin).
NOTE : The description stated above is only for information purpose. Do not take it as any medical guideline. It is essential to consult with a healthcare professional if you need any medical help.
There are several neurotransmitters that play a role in promoting wakefulness and maintaining alertness. These neurotransmitters contribute to wakefulness and alertness by stimulating specific brain regions and counteracting the effects of sleep-promoting neurotransmitters. The balance of these neurotransmitters and their interaction with sleep-promoting substances determine the overall state of alertness or sleepiness. Some examples are as follows :
Histamine: Produced mainly by the tuberomammillary nucleus (TMN) in the hypothalamus, histamine plays a significant role in maintaining alertness and wakefulness. Antihistamines, which block histamine receptors, often cause drowsiness as a side effect.
Norepinephrine: Released primarily by the locus coeruleus in the brainstem, norepinephrine is involved in attention, arousal, and vigilance. Higher levels of norepinephrine are associated with increased wakefulness.
Serotonin: Produced mainly by the raphe nuclei in the brainstem, serotonin is involved in mood regulation, appetite, and sleep. Increased serotonin levels have been associated with increased wakefulness and alertness.
Dopamine: Dopamine is a neurotransmitter involved in motivation, reward, and movement. It also plays a role in maintaining wakefulness. Certain wake-promoting medications, such as modafinil and armodafinil, are thought to work, at least in part, by increasing dopamine levels.
Acetylcholine: Acetylcholine is involved in learning, memory, and attention. It is also crucial for maintaining wakefulness and promoting rapid eye movement (REM) sleep. The basal forebrain and the brainstem are two primary sources of acetylcholine in the brain.
Orexin (hypocretin): Produced by neurons in the lateral hypothalamus, orexin is a key neurotransmitter that regulates the sleep-wake cycle. It promotes wakefulness and stabilizes the sleep-wake cycle by coordinating the activity of other neurotransmitter systems.
NOTE : Why I get difficulties with sleeping when I got medication for demantia ?
Many of the medication for dematia affects functionality of some neurotransmitter. If the medication functions same as or similar to those transmitters that are involved in maintaining wakefulness, you may get difficulties with sleeping when you take the medication.
- An example is cholinesterase inhibitors. It works by inhibiting the enzyme acetylcholinesterase, which breaks down acetylcholine. This inhibition results in increased levels of acetylcholine in the brain, which can help improve cognitive function and alleviate some of the symptoms of dementia. However, because acetylcholine is also involved in wakefulness, increased acetylcholine levels due to cholinesterase inhibitors can potentially interfere with sleep in some individuals.
Common cholinesterase inhibitors used to treat dementia include donepezil (Aricept), rivastigmine (Exelon), and galantamine (Razadyne).
There are some medications and substances can help promote wakefulness and alertness. Some common examples are:
Caffeine: Caffeine is a natural stimulant found in coffee, tea, chocolate, and some soft drinks. It works by blocking adenosine receptors, which helps counteract sleepiness and increase alertness.
Modafinil (Provigil) and Armodafinil (Nuvigil): These are prescription medications used to treat excessive daytime sleepiness in conditions like narcolepsy, obstructive sleep apnea, and shift work sleep disorder. They are thought to work by increasing dopamine levels and affecting other neurotransmitters, such as histamine and orexin.
Amphetamines: Stimulants like amphetamine (Adderall) and methylphenidate (Ritalin) are prescribed to treat attention deficit hyperactivity disorder (ADHD) and narcolepsy. They increase the levels of dopamine and norepinephrine in the brain, which helps improve attention, focus, and wakefulness.
Ephedrine and Pseudoephedrine: These are decongestants found in some over-the-counter cold and allergy medications. They have stimulant properties and can increase alertness, though their primary use is to relieve nasal congestion.
Nicotine: Nicotine, found in tobacco products, is a stimulant that increases alertness and can have a temporary awakening effect.
Guarana: Guarana is a plant native to the Amazon region, and its seeds contain high levels of caffeine. It is often used in energy drinks and supplements as a natural stimulant to increase alertness and reduce fatigue.
NOTE : The description stated above is only for information purpose. Do not take it as any medical guideline. It is essential to consult with a healthcare professional if you need any medical help.
NO. The sleep cycle or circadian rhythm is primarily regulated by the brain, but it also involves peripheral clocks in cells and tissues throughout the body. The circadian rhythm orchestrates various physiological processes to ensure optimal functioning and maintain overall health.
While the brain plays a crucial role in maintaining the circadian rhythm, it is not the only component involved. Peripheral clocks, which are present in virtually all cells and tissues in the body, also play a role in maintaining circadian rhythms. These peripheral clocks are regulated by a set of clock genes, which interact in a feedback loop to generate daily oscillations in gene expression.
The circadian rhythm influences various physiological processes in the body, including hormone production, body temperature, metabolism, and immune function. For instance, the pineal gland, located in the brain, produces melatonin, a hormone that helps regulate the sleep-wake cycle. Melatonin production is triggered by darkness and inhibited by light, with levels typically rising in the evening and decreasing in the early morning hours.
Following illustration shows the master clock (SCN) are related to other body parts and physiological system across the entire body.
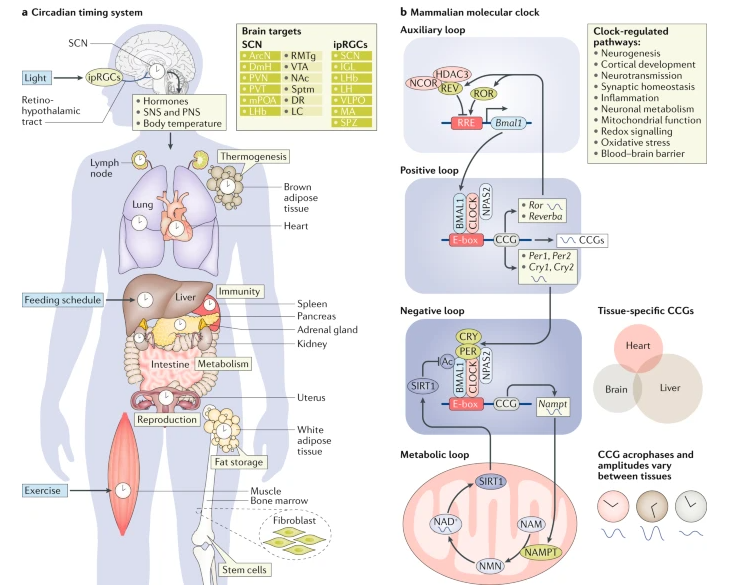
Image Source : Rhythms of life: circadian disruption and brain disorders across the lifespan
As illustrated above, the SCN plays a crucial role in coordinating the circadian rhythms of various bodily systems, ensuring optimal functioning and overall health. By synchronizing peripheral clocks through neural, hormonal, and metabolic signals, the SCN helps maintain the daily oscillations in a wide range of physiological processes.
The SCN coordinates the circadian rhythms of these various systems by synchronizing peripheral clocks through a combination of neural, hormonal, and metabolic signals. For instance, the SCN sends signals to the pineal gland to regulate melatonin production, which in turn influences peripheral clocks. Additionally, the SCN influences the release of cortisol, a hormone that helps regulate metabolism and immune function and affects circadian rhythms in various tissues.
The SCN influences the circadian rhythm of the respiratory system, affecting lung function, airway resistance, and inflammation. Circadian variations in lung function can impact the severity of respiratory diseases like asthma, with symptoms often worsening at night.
What are the Key aspects of the Lung's circadian rhythm ?
The circadian rhythm also plays a crucial role in lung function, orchestrating various pulmonary processes to align with the body's sleep-wake cycle and activity levels. Here’s how the circadian rhythm affects the lungs:
- Airway Resistance: The airways in the lungs exhibit circadian variations in resistance, typically showing higher resistance during the night. This is one reason why symptoms of respiratory diseases like asthma can worsen at night.
- Pulmonary Function: Measures of pulmonary function, such as forced expiratory volume (FEV1) and peak expiratory flow rate, demonstrate circadian fluctuations. These measures tend to be lower during the early morning hours and improve throughout the day, peaking in the afternoon.
- Gas Exchange Efficiency: The efficiency of gas exchange, the process by which oxygen is absorbed into the blood and carbon dioxide is removed, can vary throughout the day. This efficiency is crucial for maintaining optimal blood oxygen levels and can impact overall energy levels and performance.
- Inflammatory and Immune Responses: Inflammatory responses in the lungs are influenced by the circadian clock, which can affect the severity of inflammatory lung conditions. For instance, the release of certain inflammatory mediators peaks at night, potentially exacerbating respiratory symptoms.
- Surfactant Production: Pulmonary surfactant, which reduces surface tension in the alveoli and prevents lung collapse, is produced in a circadian manner. Its production typically increases during the day, aligning with higher breathing volumes and activity levels.
- Mucociliary Clearance: The process of mucociliary clearance, which helps remove mucus and pathogens from the respiratory tract, is influenced by the circadian rhythm. This process is more effective during the day, which helps in protecting the lungs from infections and maintaining clear airways.
- Hormonal Regulation: Hormones that affect lung function, including cortisol and epinephrine, are released according to a circadian pattern. These hormones can modulate immune responses, airway tone, and pulmonary vascular resistance.
Why is the Circadian Rhythm of the Lung Important?
The circadian rhythm of the lung is important for maintaining optimal respiratory function and overall health. Disruptions in the lung's circadian rhythm, such as those caused by shift work, jet lag, or sleep disorders, have been linked to various respiratory problems, including asthma, chronic obstructive pulmonary disease (COPD), and sleep apnea.
The liver has its own circadian clocks, which are synchronized by the SCN. These clocks regulate various liver functions, including metabolism, detoxification, and bile production. Disruptions in liver circadian rhythms can contribute to metabolic disorders, such as fatty liver disease and insulin resistance.
What are the Key aspects of the liver's circadian rhythm ?
The circadian rhythm significantly influences liver function, aligning metabolic processes with the body’s sleep-wake cycle and feeding patterns. Here’s how the circadian rhythm affects the liver:
- Metabolism and Detoxification: The liver plays a central role in metabolizing carbohydrates, proteins, and lipids. Its enzymatic activities fluctuate throughout the day to optimize energy use when it’s needed most. For instance, enzymes involved in glucose metabolism are more active during the day in diurnal creatures like humans, which helps manage blood sugar levels according to activity patterns.
- Protein Synthesis: The synthesis of various proteins, including those involved in blood clotting and transport, is regulated on a circadian basis. This ensures that protein production is synchronized with the body's needs at different times of the day.
- Cholesterol Regulation: The liver's production of cholesterol, a critical component of cell membranes and a precursor for steroid hormones, is subject to circadian control. This includes the synthesis of bile acids from cholesterol, which is crucial for fat digestion and is typically synchronized with meal times.
- Drug Metabolism: The liver’s ability to metabolize drugs is also influenced by the circadian clock. The expression of cytochrome P450 enzymes, which play a major role in drug metabolism, varies throughout the day. This can affect the pharmacokinetics and pharmacodynamics of medications, influencing their effectiveness and toxicity.
- Hormone Production: The liver is involved in the regulation and production of several hormones. For example, it produces insulin-like growth factor 1 (IGF-1) in a circadian pattern, crucial for regulating growth and development.
- Fatty Acid Oxidation: The liver’s role in breaking down fatty acids is also timed to the body’s energy needs, increasing during fasting states like sleep.
- Regeneration: Liver cells have the remarkable ability to regenerate, and this process is tightly linked to circadian rhythms. The timing of cell division and DNA repair mechanisms are optimized to occur when dietary toxins are lowest, typically during rest periods.
Why is the Circadian Rhythm of the Liver Important?
The circadian rhythm of the liver is crucial for maintaining metabolic homeostasis and overall health. It allows the liver to anticipate and adapt to changes in energy demands and nutrient availability throughout the day. Disruptions in the liver's circadian rhythm, such as those caused by shift work, irregular eating patterns, or sleep deprivation, can lead to various metabolic disorders, including obesity, fatty liver disease, and type 2 diabetes.
The SCN controls the circadian rhythm of blood pressure and heart rate, with both typically rising during the day and falling at night. Disruptions in this rhythm can contribute to cardiovascular diseases, such as hypertension and heart failure.
What are the Key aspects of the Heart's circadian rhythm ?
The circadian rhythm profoundly influences cardiac function, aligning heart activity with daily physiological demands and rest periods. Here’s how the circadian rhythm affects the heart:
- Heart Rate and Blood Pressure: Heart rate and blood pressure exhibit clear circadian patterns, generally being lower during sleep and rising during waking hours. This pattern prepares the body for increased activity during the day and conserves energy at night. Blood pressure typically begins to rise a few hours before waking, known as the "morning surge," which can be a risk factor for heart attacks and strokes in susceptible individuals.
- Cardiac Metabolism: The heart's metabolism of glucose and fatty acids is subject to circadian influences, optimizing energy use depending on the time of day. For instance, the heart might predominantly use fatty acids for energy during fasting states (such as overnight) and switch to glucose during times of higher activity.
- Electrophysiology: The electrical properties of the heart, which govern the heart rate and rhythm, also follow a circadian pattern. Variations in ion channel function and cardiac conduction speed throughout the day can influence heart rhythm and are thought to be one reason why incidents of arrhythmias may vary between day and night.
- Endothelial Function: The function of the endothelium, the inner lining of blood vessels, shows circadian variation, affecting vascular tone and reactivity. This is important for regulating blood flow and maintaining blood pressure levels throughout the day.
- Thrombosis Risk: The propensity for blood clot formation fluctuates with the circadian clock. For example, platelet aggregability is higher in the morning, which may contribute to the increased incidence of cardiovascular events like myocardial infarction and stroke during these hours.
- Hormonal Influence: Hormones that influence cardiovascular function, such as cortisol, adrenaline, and noradrenaline, are released in a circadian pattern. These hormones help prepare the body for the day's activities and mediate stress responses.
- Gene Expression: Many genes involved in cardiovascular function are regulated on a circadian basis. This includes genes controlling lipid metabolism, blood pressure regulation, and inflammatory responses within the cardiovascular system.
Why is the Circadian Rhythm of the Heart Important?
The circadian rhythm of the heart is essential for maintaining cardiovascular health and optimizing cardiac function throughout the day. Disruptions in the heart's circadian rhythm, such as those caused by shift work, jet lag, or sleep disorders, have been linked to increased risk of cardiovascular diseases, including hypertension, myocardial infarction (heart attack), and stroke.
The gastrointestinal system, including the intestine, has its own peripheral clocks that regulate various functions, such as nutrient absorption, motility, and secretion of digestive enzymes. The SCN synchronizes these peripheral clocks, and disruptions in the circadian rhythm can lead to gastrointestinal disorders, such as irritable bowel syndrome and inflammatory bowel disease.
What are the Key aspects of the Intestine's circadian rhythm ?
The circadian rhythm significantly influences the functioning of the intestine, orchestrating various digestive and absorptive processes in alignment with the body’s sleep-wake cycles and feeding habits. Here are some key details on how the circadian rhythm affects the intestine:
- Motility: Intestinal motility, which refers to the contractions of the muscular walls that mix and propel contents forward, exhibits a clear circadian pattern. It tends to be higher during the day to coincide with eating and lower at night, which helps in maintaining a rhythm that aligns with our eating habits and promotes efficient digestion and nutrient absorption.
- Enzyme Secretion: The secretion of digestive enzymes is also regulated by the circadian clock. For example, the production and release of enzymes like amylase, lipase, and various proteases peak during daytime or around meal times to optimize digestion.
- Nutrient Absorption: The absorption capabilities of the intestine for various nutrients, including glucose and lipids, are influenced by the circadian rhythm. This ensures that nutrient uptake is highest when food intake occurs and reduced during fasting periods.
- Microbiome Interactions: The composition and function of the gut microbiome—the community of microorganisms living in the intestine—also display circadian fluctuations. These changes can affect everything from the metabolism of dietary components to the synthesis of vitamins and the immune system modulation.
- Barrier Function: The intestinal barrier, which helps prevent the entry of harmful substances into the body while allowing nutrients to pass through, shows circadian variations in its permeability and integrity. This rhythm helps to minimize potential damage from pathogens and toxins at times when they are most likely to be encountered.
- Immune Responses: The immune activity within the gut also follows a circadian rhythm, with certain immune functions being upregulated during the day and others during the night. This cycling helps prepare the gut to deal with potential threats, such as infections or the influx of foreign substances during meals.
- Hormonal Regulation: Various hormones that influence appetite, digestion, and satiety, such as ghrelin, leptin, and insulin, are regulated according to the circadian clock. This regulation helps coordinate food intake with metabolic needs and energy utilization.
- Stem cell proliferation: The intestine has a high turnover rate, with cells continuously being replaced by new ones generated from stem cells. The proliferation of intestinal stem cells follows a circadian rhythm, with increased activity observed during the resting phase.
Why is the Circadian Rhythm of the Intestine Important?
The circadian rhythm of the intestine is crucial for maintaining optimal digestive function and overall health. It allows the intestine to anticipate and adapt to changes in food intake and nutrient availability throughout the day. Disruptions in the intestine's circadian rhythm, such as those caused by shift work, irregular eating patterns, or jet lag, have been linked to various gastrointestinal disorders, including irritable bowel syndrome (IBS), inflammatory bowel disease (IBD), and obesity.
Skeletal muscles have their own circadian clocks that regulate various aspects of muscle function, including metabolism, contractility, and repair. The SCN synchronizes these clocks, and disruptions in muscle circadian rhythms can contribute to muscle weakness, impaired performance, and increased risk of injury.
What are the Key aspects of the Muscle's circadian rhythm ?
The circadian rhythm significantly impacts muscle function and metabolism, influencing performance, repair, and overall muscle health. Here’s how the circadian rhythm affects muscle tissue:
- Muscle Performance: Muscle strength and endurance exhibit a clear circadian pattern, typically peaking in the late afternoon when body temperature is highest. This timing aligns with optimal muscle function and reduced injury risk, suggesting that the time of day can influence workout effectiveness and safety.
- Protein Synthesis and Muscle Repair: Muscle growth and repair are strongly influenced by circadian rhythms, with protein synthesis peaking during sleep. This is partly driven by the release of growth hormone and other anabolic hormones during certain sleep stages, which are essential for muscle repair and growth.
- Energy Metabolism: Muscle cells adjust their preference for energy substrates (carbohydrates, fats) across the circadian cycle. For instance, insulin sensitivity and glucose uptake by muscle cells are higher during the day than at night, aligning with eating patterns and physical activity levels.
- Mitochondrial Function: The mitochondria in muscle cells, which are responsible for energy production, also operate on a circadian cycle. Their efficiency in generating energy can vary throughout the day, affecting how muscles perform and recover from exercise.
- Oxidative Stress and Antioxidant Defense: The production of reactive oxygen species (ROS) in muscles and the corresponding antioxidant defenses are under circadian control. This helps balance the beneficial and potentially harmful effects of ROS, which are involved in signaling but can also contribute to cellular damage if not regulated.
- Inflammation and Immune Responses: Circadian rhythms regulate the inflammatory responses within muscle tissue, which is crucial after injury or during diseases such as muscular dystrophy. Proper timing of these responses helps in efficient repair without excessive damage.
- Gene Expression: Many genes involved in muscle function, including those for muscle development, metabolic enzymes, and structural proteins, are expressed in a circadian manner. This gene expression regulation ensures that muscle physiology is optimized for the body's varying needs throughout the day and night.
Why is the Circadian Rhythm of the Muscle Important?
The circadian rhythm of skeletal muscle is essential for maintaining muscle function, optimizing athletic performance, and promoting muscle growth and repair. Disruptions in the muscle's circadian rhythm, such as those caused by shift work, jet lag, or sleep deprivation, have been linked to decreased muscle strength, impaired exercise performance, and increased risk of muscle injuries.
The kidney has its own circadian clock, synchronized with the central circadian pacemaker (SCN) in the hypothalamus. Morning involves increased urine production and electrolyte excretion, preparing the body for daily activities. Daytime maintains steady urine production and electrolyte balance to support the body. At night, water and electrolyte conservation occurs due to increased ADH and aldosterone levels, resulting in reduced urine output. This 24-hour cycle ensures
optimal
kidney function, hydration, and electrolyte homeostasis.
How Does the Circadian Rhythm Affect Kidney Function?
The circadian rhythm of the kidney is regulated by the body's internal clock, located in the suprachiasmatic nucleus (SCN) of the brain. The SCN receives signals from the environment, such as light and darkness, and synchronizes the body's physiological processes accordingly.
In the kidneys, the circadian clock controls the expression of clock genes, which in turn regulate various renal functions. For example, the expression of aquaporin-2 (AQP2), a water channel protein involved in urine concentration, peaks at night, leading to increased water reabsorption and reduced urine output during sleep.
The circadian rhythm of the kidney is an intriguing aspect of how our bodies adapt physiologically to the 24-hour day/night cycle. Here are some key details:
- Variation in Filtration Rate: The glomerular filtration rate (GFR), which measures how well the kidneys filter blood, varies over the course of the day. It is typically lower during nighttime sleep. This natural fluctuation is thought to be adaptive, helping to conserve energy and manage fluid balance while we sleep.
- Changes in Urine Production: Corresponding to the changes in GFR, the rate of urine production also decreases at night. This helps in reducing the frequency of waking up to urinate, thus promoting uninterrupted sleep.
- Hormonal Regulation: Various hormones contribute to the circadian rhythm of kidney function. For example, aldosterone, which helps regulate sodium and water balance, and antidiuretic hormone (ADH), also known as vasopressin, which helps control the body's water balance by affecting the kidneys, both have patterns that align with the circadian rhythm.
- Molecular Clock: Kidneys have intrinsic molecular clocks that contribute to their circadian rhythm. These molecular clocks regulate various genes that control processes like sodium and potassium handling, as well as water reabsorption.
- Impact on Drug Metabolism: The circadian rhythm of the kidney affects the pharmacokinetics of various drugs. For instance, medications that are metabolized or excreted by the kidneys might have different efficiencies or side effects depending on the time of day they are administered.
- Health Implications: Disruptions in the circadian rhythms of kidney function can have health consequences. For example, shift workers, who have altered sleep cycles, often experience disruptions in these rhythms, which can affect kidney function and overall fluid balance.
Why is the Circadian Rhythm of the Kidney Important?
The circadian rhythm of the kidney plays a critical role in maintaining optimal renal function and overall health. This natural biological cycle helps regulate various kidney functions, including blood filtration, urine production, and hormone secretion, which are pivotal for body fluid and electrolyte balance. Disruptions in the circadian rhythm, such as those caused by shift work, jet lag, or sleep disorders, can lead to several adverse health conditions:
- Hypertension (High Blood Pressure): Proper circadian regulation helps maintain blood pressure levels by controlling the secretion of hormones like aldosterone and vasopressin, which manage fluid balance and vascular resistance. Disruption of these cycles can lead to elevated blood pressure at night, increasing the risk of developing hypertension.
- Chronic Kidney Disease (CKD): Irregularities in circadian rhythms can affect kidney function over time, potentially leading to a gradual loss of kidney function. This can be exacerbated by prolonged high blood pressure and diabetes, which are also influenced by circadian misalignments.
- Kidney Stones: The circadian rhythm influences urine concentration and excretion rates. Disruption can lead to higher concentrations of minerals and salts in the urine at times when the body isn’t prepared to dilute these substances efficiently, increasing the risk of kidney stone formation.
- Renal Fibrosis: This condition involves the scarring of kidney tissue, often resulting from chronic inflammation or injury. The repair processes of the kidney are also under circadian control, including the activity of fibroblasts and the inflammatory response. Disruption in these processes can accelerate the progression of renal fibrosis.
- Metabolic Impact: The kidneys' ability to metabolize and excrete drugs and metabolites also follows a circadian pattern. Disruption can lead to inefficient drug metabolism, affecting the efficacy and toxicity of medications.
The immune system is also under circadian control, with the SCN influencing the timing of immune cell production, activation, and migration. Circadian variations in immune function can impact the severity and duration of infections, as well as the response to vaccinations.
How does the circadian rhythm affect the immune system?
The circadian rhythm significantly influences the immune system, coordinating its activities to optimize defense mechanisms while aligning with the body’s overall energy utilization and conservation cycles. Here are some critical aspects of how the circadian rhythm affects the immune system:
- Immune Cell Traffic: The circulation of immune cells, such as leukocytes, is subject to circadian variations. The migration of immune cells between different tissues, such as the blood, lymph nodes, and peripheral organs, follows a circadian pattern.
- The number of lymphocytes (a type of white blood cell) circulating in the blood peaks at night, potentially enhancing immune surveillance during sleep.
- Levels of certain white blood cells in the bloodstream peak during the day to increase potential interaction with pathogens when we are more active and decrease at night, allowing for rest and recovery.
- Inflammatory Responses: The intensity and timing of inflammatory responses are influenced by the circadian clock. For instance, inflammatory markers often show higher levels during the night or early morning, which is why symptoms of chronic inflammatory diseases like rheumatoid arthritis tend to worsen at these times.
- Cytokine Release: Cytokines are signaling molecules that mediate and regulate immunity, inflammation, and hematopoiesis. The production of various cytokines is under circadian control, impacting how the body responds to infection and inflammation throughout the day. The production of many cytokines, including pro-inflammatory cytokines like tumor necrosis factor-alpha (TNF-α) and interleukin-6 (IL-6), exhibits a circadian rhythm, with higher levels observed at night or during
the resting phase.
- Immune cell function:The activity and responsiveness of immune cells can vary depending on the time of day. For instance, some studies suggest that natural killer (NK) cells, which are important for fighting viral infections and tumors, may be more active at night.
- Antigen Presentation: The process by which cells display antigen on their surface to be recognized by T-cells can also be influenced by the circadian clock. This can affect how effectively the immune system identifies and reacts to pathogens.
- Vaccine Efficacy: Research has shown that the timing of vaccination can affect the immune response. Vaccinations administered in the morning have sometimes been found to produce a more robust immune response compared to those given in the afternoon or evening.
- Wound Healing: The body's ability to repair tissue and heal wounds is influenced by circadian rhythms, with certain reparative processes peaking during sleep. This is partially due to changes in the release of growth factors and other healing-related molecules.
- Infection Susceptibility: Susceptibility to infections can vary with the time of day, likely due to fluctuations in immune function. For example, studies have suggested that the body may be more vulnerable to viral infections at certain times due to the varying levels of immune cells and antiviral molecules.
Why is the circadian rhythm of the immune system important?
The circadian rhythm of the immune system plays a crucial role in optimizing immune function and maintaining overall health. It allows the immune system to anticipate and respond to potential threats more effectively at different times of the day.
Disruptions in the circadian rhythm, such as those caused by shift work, jet lag, or sleep deprivation, can negatively impact immune function. These disruptions have been linked to increased susceptibility to infections, chronic inflammation, and autoimmune diseases.
The circadian rhythm plays a pivotal role in regulating the metabolic rate, ensuring that the body's energy usage and storage are efficiently synchronized with daily patterns of activity and rest. This biological clock influences various aspects of metabolism, from the basal metabolic rate, which varies between day and night, to the specific processing of glucose and lipids according to time of day. The rhythm extends its reach to hormonal influences that orchestrate these processes, impacting
everything from insulin sensitivity to hunger and thermogenesis. Understanding these rhythmic patterns is crucial for maintaining metabolic health and preventing related disorders.
How Does the Circadian Rhythm Affect Metabolic Rate?
The circadian rhythm profoundly influences metabolic rate, coordinating the body's energy usage and storage to align with daily patterns of activity and rest. The circadian rhythm significantly affects metabolic rate, which is the rate at which your body expends energy. This influence is primarily mediated by the body's internal clock, the suprachiasmatic nucleus (SCN), located in the brain.
Here's a detailed look at how the circadian rhythm affects metabolic rate:
- Energy Expenditure: The body's basal metabolic rate (BMR), which is the amount of energy expended while at rest, fluctuates according to the circadian cycle. BMR typically increases during the daytime when activity levels are higher and decreases at night during sleep, reflecting the body's reduced need for energy.
- Glucose Metabolism: The metabolism of glucose is closely tied to the circadian clock. Insulin sensitivity and the production of insulin by the pancreas show significant circadian variation, with peak sensitivity typically occurring in the afternoon and early evening. This timing helps manage blood glucose levels more efficiently during periods of food intake and activity.
- Lipid Metabolism: The processing of fats is also under circadian control. Lipolysis, the breakdown of fat stores into fatty acids, is generally higher during the night to provide energy during fasting states. Conversely, lipid synthesis is more active during the day, particularly after meals when dietary fats are available.
- Hormonal Influence: Hormones that regulate metabolism, such as cortisol, growth hormone, and thyroid hormones, are released in a circadian pattern. Cortisol, for instance, peaks in the early morning, helping to stimulate glucose production for energy as we wake up.
- Appetite and Digestion: The circadian rhythm affects hunger and satiety hormones, such as ghrelin and leptin, influencing when and how much we eat. Ghrelin, which stimulates appetite, typically peaks just before meals, while leptin, which signals satiety, rises after eating.
- Thermogenesis: The production of heat in the body, a process called thermogenesis, is influenced by the circadian clock. It is usually higher during the day, contributing to a higher metabolic rate when the body is more active.
- Gene Expression: Many genes involved in metabolism are expressed in a circadian manner. This includes genes that regulate the enzymes responsible for breaking down carbohydrates and fats, as well as those involved in energy production within cells.
How Disruptions in the circadian rhythm and metabolic health affect health in general ?
Disruptions in the circadian rhythm, such as those caused by shift work, jet lag, or sleep deprivation, can have detrimental effects on metabolic health. These disruptions have been linked to:
- Decreased glucose tolerance: The body's ability to process sugar efficiently is impaired, increasing the risk of insulin resistance and type 2 diabetes.
- Increased fat storage: Disrupted circadian rhythms can promote fat accumulation, leading to obesity and related metabolic disorders.
- Altered hormone levels: Cortisol, insulin, and other metabolic hormones may become dysregulated, further contributing to metabolic dysfunction.
The sleep cycle (circadian rhythm) can change over the course of a person's life. These changes are often related to different stages of development and aging. Several factors contribute to changes in sleep patterns and circadian rhythms across the lifespan, including alterations in the production and regulation of sleep-related hormones (such as melatonin), changes in brain structure and function, and lifestyle factors (such as work schedules and exposure to artificial light). It is essential
to recognize and adapt to these changes in sleep patterns to maintain overall health and well-being.
Following is plot showing the changes of Melatonin, Core body temperature, Cortisol during 24 hours and over the life time. Just with a first glimse, I see the patterns that many people say just based on their experience or observation.
- Babies sleep most of the time
- Children sleep early and wake up relatively early.
- Teenagers (adolescent) have difficulties waking up early (e.g, school time) and sleep late
- Elderly people sleep early and wake up very early. They have the least amount of sleep
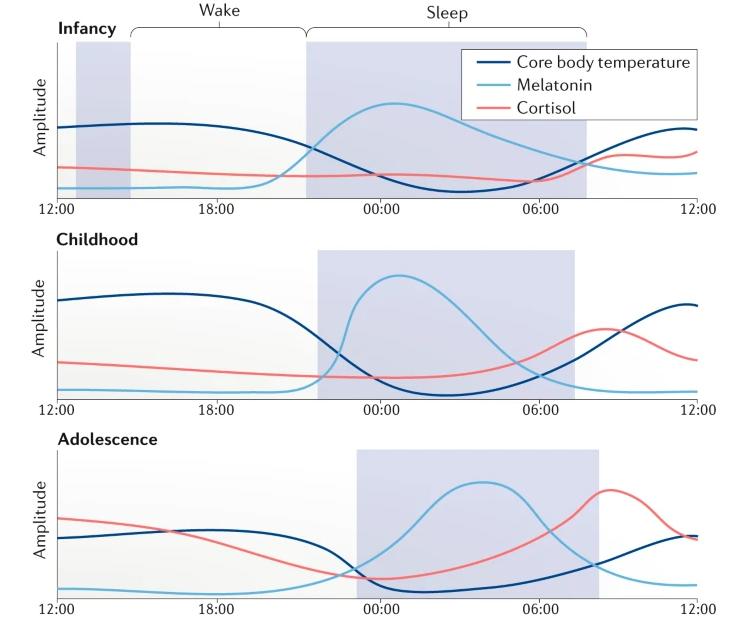
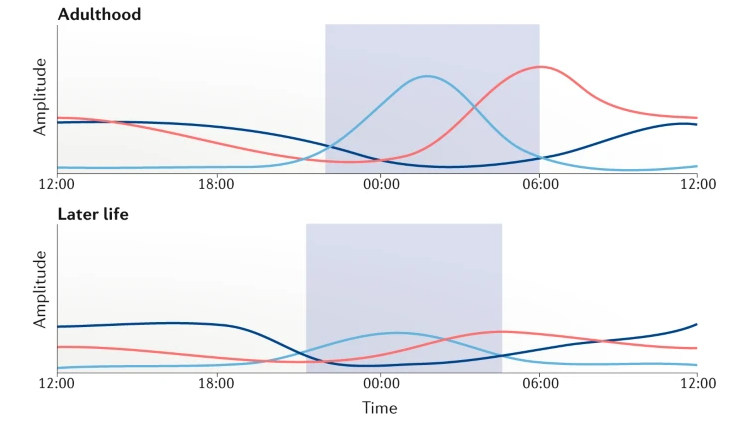
Image Source : Rhythms of life: circadian disruption and brain disorders across the lifespan
Following is the description on the pattern in terms of melatonin, core body temperature, cortison within 24 hours for the period of infancy, childhood, adolsescence, Adulthood, Later life.
Infancy:
- Melatonin: As the infant's sleep pattern consolidates, melatonin release becomes more regular, with levels rising in the evening and decreasing in the early morning hours. However, this pattern may not be fully established, resulting in irregular sleep-wake cycles.
- Core body temperature: The circadian rhythm of core body temperature is not well-developed in infants, and temperature fluctuations may be less pronounced and more variable throughout the day and night.
- Cortisol: The circadian rhythm of cortisol secretion gradually emerges during infancy, with increasing morning peaks and lower evening levels.
Childhood:
- Melatonin: Melatonin levels rise in the evening, promoting sleepiness, and decrease in the early morning hours, corresponding to the sleep-wake cycle. The timing of melatonin release is typically consistent with the child's sleep schedule.
- Core body temperature: In children, the circadian rhythm of core body temperature is more established, with higher temperatures during the day and lower temperatures at night.
- Cortisol: Cortisol levels peak in the morning, providing an energy boost, and gradually decline throughout the day, reaching their lowest levels in the evening and at night.
Adolescence:
- Melatonin: During adolescence, melatonin release is often delayed, leading to later bedtimes and wake times. Levels rise later in the evening and decrease later in the morning compared to childhood.
- Core body temperature: The circadian rhythm of core body temperature in adolescents is still present, but the lowest temperature may occur later in the night compared to children and adults.
- Cortisol: The diurnal pattern of cortisol secretion remains consistent during adolescence, with peak levels in the morning and lower levels in the evening.
Adulthood:
- Melatonin: In adults, melatonin production and release follow a stable pattern, with levels increasing in the evening, promoting sleepiness, and decreasing in the early morning hours, promoting wakefulness.
- Core body temperature: The circadian rhythm of core body temperature in adults is well-established, with higher temperatures during the day and lower temperatures at night, typically reaching the lowest point in the early morning hours.
- Cortisol: The diurnal pattern of cortisol secretion in adults is consistent, with peak levels in the morning, providing an energy boost, and a gradual decline throughout the day, reaching their lowest levels in the evening and at night.
Later life:
- Melatonin: Melatonin production tends to decline with age, which can contribute to sleep disturbances and difficulty falling asleep in older adults. Levels may rise earlier in the evening and decrease earlier in the morning compared to younger adults.
- Core body temperature: The circadian rhythm of core body temperature may be less pronounced in older adults, with a reduced amplitude of temperature fluctuations and a potential shift towards earlier temperature peaks and troughs.
- Cortisol: In older adults, the diurnal pattern of cortisol secretion can become less distinct, with less variation between morning and evening levels. This change may contribute to age-related sleep disturbances and altered energy levels.
NOTE : Why cortisol is important to check during the circadian cycle ?
Cortisol plays an essential role in the sleep-wake cycle and overall circadian rhythm because it helps regulate various physiological processes, including metabolism, immune function, and stress response. Cortisol plays a crucial role in the sleep-wake cycle and overall circadian rhythm by regulating energy levels, metabolism, immune function, and the body's response to stress.
- Energy and alertness: Cortisol is often referred to as the "stress hormone" because it is released in response to stress, helping the body cope with challenging situations. Cortisol levels are highest in the morning, providing an energy boost and promoting alertness, which helps individuals wake up and start their day. Throughout the day, cortisol levels gradually decrease, reaching their lowest point in the evening and at night, allowing the body to wind down and prepare for
sleep.
- Metabolic function: Cortisol influences glucose metabolism by promoting the breakdown of glycogen into glucose, providing energy for the body. This process is crucial for maintaining stable blood sugar levels and ensuring that the body has enough energy to function throughout the day. By regulating metabolism, cortisol plays a role in maintaining the sleep-wake cycle and overall circadian rhythm.
- Immune function: Cortisol modulates the immune system by suppressing inflammation and altering the activity of immune cells. Its diurnal variation helps regulate the timing of immune responses, with higher cortisol levels during the day suppressing inflammation and promoting tissue repair, while lower levels at night allow the immune system to respond more robustly to infections and other challenges.
- Interaction with other hormones: Cortisol interacts with other hormones involved in the sleep-wake cycle, such as melatonin. For example, high cortisol levels in the evening can interfere with melatonin production and delay the onset of sleep. Conversely, low cortisol levels in the morning can make it more difficult to wake up and start the day.
- Stress response: Cortisol helps the body cope with stress by mobilizing energy resources, increasing heart rate, and heightening alertness. However, chronic stress can lead to dysregulation of cortisol secretion, with consistently elevated levels disrupting the sleep-wake cycle and contributing to sleep disturbances, fatigue, and other health problems.
Reference
- Sleep
- Circadian Rythm / Sleep-Awake Pattern
- Dream
YouTube
|
|